RESEARCH
(2) Nanozymes for Biomedical Applications
Engineering bio-compatible nanomaterials to mimic the cellular antioxidant machinery has assumed priority in translation research to combat oxidative stress mediated disorders. Particularly, nanomaterials with intrinsic enzyme-like activity attract significant current interest due to their ability to replace specific enzymes in enzyme-based applications. A few nanostructured materials have been shown to mimic the activity of peroxidases, oxidase, and superoxide dismutase. The main objective of our work is to understand how the surface of nanomaterials can be engineered to perform specific reactions. We believe that the enzyme-like properties of nanomaterials (nanozymes) can be fine-tuned by controlling the size, shape and morphology, surface coating and modification and composition. There appears to be a change in the enzyme-like activity when a bulk material is converted to nanoform. Recently, our group, in collaboration with D'Silva's group has conceptualized the development of a vanadium-based nanozyme that specifically exhibits GPx activity and possesses a cytoprotective function in the cell (Vernekar, A. A. et al. Nature Commun. 2014, 5, 5301, Click here for details).
The rod-shaped vanadia (V2O5) nanowires efficiently internalize into the cell by endocytic mechanisms without altering the integrity of the biological membranes. The nanowires by themselves are not toxic to the cell and exhibit remarkable antioxidant properties by reducing the accumulated peroxides within the stressed cell. Mugesh’s group competently showed that vanadia nanowires require GSH as a co-factor for its antioxidant properties both in vitro and in live cells, and mimic GPx
(Figure 5). These nanozymes could efficiently replace GPx in the cyclic GSH-GSSG cascade and function alongside with glutathione reductase and electron donor, NADPH. Like the natural enzyme, vanadia nanowires showed substrate selectivity to hydrogen peroxide, with the formation of a peroxo species on nanowire surface being the rate determining step. Although vanadia nanowires are known to function as a haloperoxidase mimic and generate a strong oxidant hypobromous acid, its antioxidant activity is not altered even in the presence of haloperoxidase substrates.
In a nano form, V2O5 is able to reduce hydrogen peroxide without changing the oxidation state of the metal, which is unusual for a metal ion. If the metal changes its oxidation state, vanadium
Figure 4. Fine-tuning the surface chemistry for specific applications.
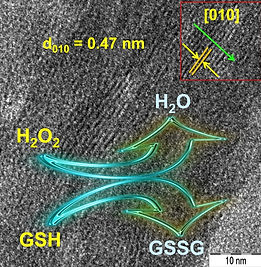
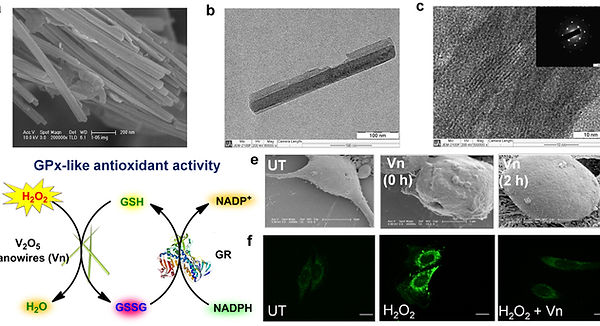
Figure 5. Nanovanadia - Turning cytotoxic vanadium into cytoprotective antioxidant: (a) SEM image of vanadium pentoxide nanowires (Vn). (b) and (c) TEM and HRTEM images of Vn showing the planes and inset reveals the crystallinity and orthorhombic crystal structure of Vn. (d) Glutathione peroxidase activity of Vn and GSH recycling by glutathione reductase. (e) SEM images of HEK293T cells untreated (UT) or treated with Vn. (f) Hydrogen peroxide scavenging activity of Vn in HeLa cells.
Funding for Research
can produce reactive oxygen species, which is what happens when vanadium is in a bulk form. Although the number of nanowires that get into a cell cannot be controlled, the amount of vanadia nanowires used is very small - in parts per million (ppm). Apparently, the inability to completely remove hydrogen peroxide turned out to be beneficial as optimum removal ensures that other biological functions remain unaffected by vanadia. Turning a cytotoxic vanadium into cytoprotective antioxidant by reducing the size of the material is certainly a novel concept. The vanadia nanozyme is of therapeutic importance in combating age-related degenerative diseases and as a delivery vehicle for various xenobiotics to reduce stress mediated side-effects.
We also reported the synthesis and glutathione peroxidase‐like enzyme mimetic activity of orthorhombic V2O5 nanozymes in four different morphologies, nanowires (VNw), nanosheets (VSh), nanoflowers (VNf), and nanospheres (VSp) and show that the activity does not correlate with their surface area. We demonstrate for the first time that the surface‐exposed crystal facets within the same crystal system can alter the H2O2 reducing ability of V2O5 nanozymes. The activity depends on the relative concentrations of H2O2 and glutathione (GSH) and at higher H2O2 concentrations, the nanospheres exhibit remarkably higher activity than that of nanowires, indicating that the crystal facets play crucial roles in the catalytic activity. The variations in the GPx‐like activity originate from the difference in the rate of formation of a V‐peroxido species on the surface. The results described herein on the modulation of redox reactions by altering the size, shape, and crystal facets may open up opportunities not only for the design and synthesis of nanomaterials with enzyme‐like activity, but also for the development of nanomaterial‐based isozymes (nanoisozymes), which essentially catalyze the same chemical reactions, but exhibit a compartment‐specific activity in biological systems Ghosh et al. Angew. Chem. Int. Ed. 2018, 57, 4510).
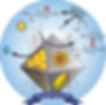
Figure 6. We coined the term “nanoisozymes” to describe how the different exposed crystal facets on V2O5 surfaces in the same crystal system mimic the redox regulation of the enzyme.
Development of Oxidase-like Nanozymes for Biomedical Applications
A few years ago, our group reported that nanomaterials can be used to functionally mimic the oxidase enzymes, a class of metalloenzymes that catalyze the oxidation of a variety of organic substrates in the presence of molecular oxygen. In the enzymatic reactions, molecular oxygen acts as an electron acceptor, which is reduced to water or hydrogen peroxide depending upon the nature of oxidase. Our experiments revealed that H2O2 is not produced during the course of the oxidase-like reactions and that MnFe2O4 NOh acts predominantly as oxidase mimic rather than as peroxidase mimic.

(a) Oxidase‐like activity in the presence of different substrates, TMB (1), ABTS (2), OPD (3), and dopamine (4). The oxidase activity was measured in the presence of TMB (40 μm) and NOh (3.89 pM) in citric acid buffer (pH 4). (b) Activity is highly pH‐dependent and the reaction is more favored at pH 4. (c) Comparison of the activity of different nanomaterials. (d) Increase in the concentration of the catalyst proportionally increases the rate of the reaction. (e) Enzyme‐like kinetics is shown by Michaelis–Menten plot.
Vernekar et al. Chem. Asian J. 2016, 11, 72-76.

Redox Modulatory Mn3O4 Nanozyme with Multi-enzyme Activity
The work in our group is aimed at the development of a potent biocompatible nanomaterial that can supplement the enhanced cellular requirement to scavenge elevated ROS levels under pathophysiological conditions. Recently, we reported that a Mn3O4 nanozyme functionally mimics three major antioxidant enzymes, that is, superoxide dismutase (SOD), catalase (CAT) and glutathione peroxidase (GPx). The redox modulatory effect of Mn3O4 plays a crucial role in protecting the cells from MPP+ induced cytotoxicity in a Parkinson disease (PD)‐like cellular model, indicating that manganese‐based nanomaterials having multi‐enzyme activity can robustly rescue the cells from oxidative damage and thereby possess therapeutic potential to prevent ROS‐mediated neurological disorders.

Figure 7. The Mn3O4 nanozyme modulates the redox state of the mammalian cells by functionally mimicking the activity of three major enzymes, superoxide dismutase (SOD), catalase (CAT) and glutathione peroxidase (GPx) and provides cytoprotection against oxidative damages caused by reactive oxygen species (ROS).
We have demonstrated for the first time that a single nanomaterial, Mn3O4 nanoflowers (Mnf ), exhibits the activity of three major structurally and functionally different antioxidant enzymes, catalase (CAT), glutathione peroxidase (GPx) and superoxide dismutase (SOD), which maintain the redox homeostasis in mammalian cells by controlling the level of reactive oxygen species (ROS). Mnf also effectively scavenges hydroxyl radicals, which are known to cause damage to biomolecules. A mechanistic investigation reveals that the mixed oxidation states of manganese (Mn2+/Mn+3), high stability against irreversible oxidation, large surface area and unusually large pore size are important for the remarkable multi‐enzyme
activity of Mnf. Interestingly, Mnf effectively internalizes into human cells and provides efficient cytoprotection in a Parkinson's disease model. This study suggests that Mnf can be considered as a potential candidate for therapeutic applications against oxidative stress‐induced neurological disorders. (Singh et al Angew. Chem. Int. Ed. 2017, 56, 14267). Further studies revealed that the nanozyme activity of Mn3O4 depends on various factors including size, morphology, surface area, and the redox properties of the metal ions. The Mn3O4 nanoflowers exhibited remarkably high activity in all three enzyme systems and the order of multienzyme activity of different morphologies was: flowers ≫ flakes > hexagonal plates ≈ polyhedrons ≈ cubes. All five nanoforms are taken up by the mammalian cells and were found to be biocompatible, with very low cytotoxicity. The activity of the most active nanoflowers was studied in primary human umbilical vein endothelial cells (HUVEC) and human pulmonary microvascular endothelial cells (hPMEC) and it was found that Mn3O4 does not reduce the level of nitric oxide (NO). This is in contrast to the effect of some of the Mn‐porphyrin‐based SOD mimetics, which are known to scavenge NO in endothelial cells. (Singh et al. Chem. Eur. J. 2018, 24, 8393).

Figure 8. The Mn3O4 nanozyme modulates the redox state of the mammalian cells by functionally mimicking the activity of three major enzymes, superoxide dismutase (SOD), catalase (CAT) and glutathione peroxidase (GPx) and provides cytoprotection against oxidative damages caused by reactive oxygen species (ROS).
Catalytic Reduction of Molecular Oxygen - Cytochrome c Oxidase Activity of Cerium Vanadate Nanozyme
Cytochrome c oxidase (CcO) is the terminal metalloenzyme in the mitochondrial electron transport chain and consumes more than 90% of the respired oxygen in mammalian cells. CcO catalyzes the four-electron reduction of oxygen to water by receiving electrons from the heme-containing protein, cytochrome c (cyt c). The structure and function of the enzyme is affected in several disease conditions, including cancer, neurodegeneration and diabetes. A decrease in the CcO activity leads to oxidative stress and mitochondrial dysfunction, which ultimately results in cellular energy crisis. As the functional unit of CcO consists of several metal prosthetic sites and protein subunits, the development of synthetic mimics for this enzyme is very challenging. In addition, the inefficient electron transfer to the active sites of the functional models leads to the release of partially reduced oxygen species (PROS) such as superoxide (O2-), peroxide (O22-) and hydroxyl radicals (·OH) that are highly toxic to the cells.[6] Although an increase in the O2- level during the oxidative phosphorylation is controlled by the superoxide dismutase (SOD) (Figure 9), a high amount of PROS can lead to mitochondrial dysfunction.


We reported for the first time that CeVO4 nanozymes effectively catalyze the four-electron reduction of molecular oxygen to water at physiological pH by functionally mimicking the mitochondrial enzyme, cytochrome c oxidase (CcO), utilizing cytochrome c as electron donor. Interestingly, the complete reduction of oxygen to water takes place without the release of any partially reduced oxygen species (PROS). In addition to its CcO-like activity, the CeVO4 nanozymes exhibit a robust superoxide dismutase (SOD)-like activity. The ability of the non-toxic CeVO4 nanozymes to functionally mimic the two crucial mitochondrial enzymes may help in developing novel nanomaterials that can maintain the mitochondrial integrity under disease conditions (Singh and Mugesh, Angew. Chem. Int. Ed. 2019, 58, 7797).
Figure 9. Top: Schematic diagram of the respiratory electron transport chain of mitochondria, indicating the role of cytochrome c oxidase (CcO) and superoxide dismutase (SOD). Bottom: CeVO4 Nanozymes Catalyze the Reduction of Dioxygen to Water without Releasing Partially Reduced Oxygen Species (PROS).
Modulation of Nitric Oxide (NO) release from S-Nitrosothiols by Cu2O Polymorphs
It is well known that nitric oxide (NO) is involved in a variety of important biological processes such as a signalling molecule in the nervous system, vasodilator in the cardiovascular system, and it is used as a key molecule by the immune system to fight against infections. However, the imbalance between the nitrosylation and denitrosylation activities of enzymes affect the NO concentration, which ultimately results in the formation of nitrosylated proteins via a covalent binding of NO to the cysteine residues of proteins. The nitrosylation of proteins leads to various deleterious effects, which include the monomerization of functionally active endothelial nitric oxide synthase (eNOS), leading to the production of superoxide species that can react with NO to generate the highly toxic peroxynitrite (ONOO−) and elevation of oxidative stress in the cells. It has been shown that nitrosylated Hsp90 inhibits the ATPase activity, which leads to the decrease in the eNOS activity. As NO is synthesized in endothelial cells by eNOS, the low level of NO due to impairment of the enzyme activity affects the endothelial function. On the other hand, elevated levels of NO cause nitrosative stress related signalling, leading to post-translational modification of many proteins, neurotoxicity and apoptosis. NO concentration above 1.0 μM results in nitrosative stress and necrotic cell death by generating a large amount of reactive nitrogen species (RNS). Therefore, the biological effects of NO are highly dependent on its concentration.
Figure 10. Crystal Facet-dependent Cu2O-mediated denitrosylation of S-nitrosothiols and release of nitric oxide (NO).

We demonstrated that Cu2O-based nanomaterials can release NO from S-nitrosothiols and the catalytic activity is morphology-dependent. This study provides the first experimental evidence that the denitrosylating activity of Cu2O nanocrystals can be altered by changing the crystal facets within the same crystal system. Surprisingly, no direct correlation between the activity and the surface area of the materials was observed. The different arrangement of the atoms on the surface is found to be responsible for the remarkable change in the denitrosylation activity of Cu2O nanomaterials having various morphologies. A detailed mechanistic investigation revealed that the higher activity of the Cu2O nanomaterial having Oh morphology as compared to that of RDh and cube is due to the presence of the {111} crystal facet in Oh, which is more efficient than the {011} and {001} facets present in RDh and cube, respectively, in the denitrosylation of S-nitrosothiols. Further, the Cu2O Oh nanocrystals having {111} facets may be employed for a quick release of NO, whereas the RDh morphology having the {011} facets will be useful for a slow and sustained release of NO. Therefore, the facet-dependent denitrosylation activity described in this paper may find potential applications in modulating the NO bioavailability under disease conditions where the physiological NO signaling is severely impaired. For example, the decrease in the NO levels in endothelial cells is known to cause endothelial dysfunction, leading to several vascular diseases. The materials that can improve the levels of NO in these cells are likely to be potential candidates for the treatment of vascular disorders.