RESEARCH
(4) Halogen bond-mediated Cell Membrane Transport
The efficient delivery of therapeutically active small molecules into mammalian cells is important for the development of new drugs. The transport of chemical compounds into cells is controlled by the plasma membrane, which consists of various receptors and transporters that mediate the intracellular delivery of biologically active compounds. Although small gaseous molecules such as oxygen, carbon dioxide, and nitric oxide can cross the lipid bilayer by simple diffusion, larger hydrophilic molecules have poor membrane permeability, and, therefore, these molecules require specific transporters such as transmembrane proteins for their entry into the cell. While the transport of small molecules into the cells has been improved by using various delivery systems such as liposomes and nanoparticles, the inefficiency of the delivery systems and side effects associated with the drug carriers are major challenges in biomedical applications. For this reason, many larger molecules such as proteins have been developed for extracellular targets. Recent studies focused on the development of cationic cell‐penetrating peptides (CPPs), antibodies, nanoparticles, receptor ligands, virus‐like particles, and supercharged proteins (SCPs) for the delivery of functional macromolecules.
Improving the Cellular Delivery by Halogen Substitution
Many drugs and drug candidates in clinical development have halogen atoms, particularly chlorine and bromine. However, it is not clear whether the presence of halogen atoms in therapeutically useful compounds improves their cellular uptake. Recently, our group reported that the introduction of halogen, particularly iodine, atoms to naphthalimide‐based fluorescent molecules increases the transport across the plasma membrane (Ungati et al. Angew. Chem. Int. Ed. 2018, 57, 8989). In compounds 1–5, the push–pull mechanism involving the −NMe2 group acting as the electron donor and the substituted phenyl group acting as the electron acceptor is responsible for the fluorescence (Figure 11A). The fluorescence intensity depends on the substituents at the phenolic ring. As expected, a decrease in the fluorescence intensity
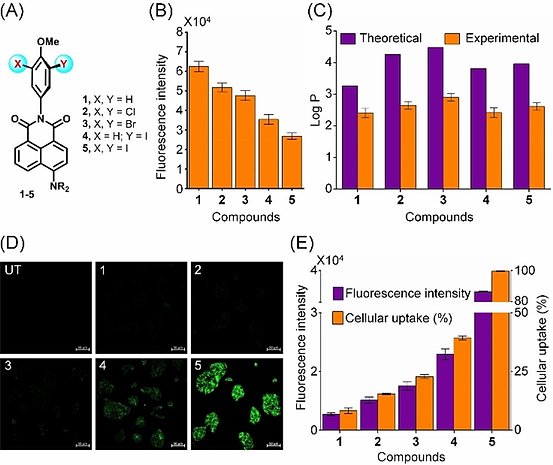
was observed upon introduction of chlorine, bromine, and iodine atoms (compounds 2–5) due to quenching of the fluorescence by the heavy atoms (Figure 11B). The experimental and theoretical investigations on the lipophilic nature of the compounds indicated that there is no major change in the lipophilicity upon introduction of the heavier halogen, particularly iodine, atoms (Figure 11C).
Figure 11. (A) Chemical structures of the naphthalimide‐based fluorescent molecules 1–5. (B) The relative fluorescence intensity for compounds 1–5 in water. (C) The lipophilicity of 1–5 given in terms of their logP values. (D) Confocal microscopy images of HepG2 treated with compounds 1–5 (10 μM ) for 30 min (UT: untreated). Scale bar represents 50 μM. (E) The fluorescence measured by a plate reader after 30 min of treatment of HepG2 cells with compounds 1–5 (10 μM) and the total uptake of compounds 1–5 by HepG2 cells.
Funding for Research
The cellular uptake of the fluorescent molecules 1–5 has been studied in HepG2 (human liver carcinoma) cells by using laser scanning microscopy and fluorescence microplate reader techniques. The fluorescence observed for the cells treated with compound 1 was found to be very low (Figure 11D), indicating a poor cellular uptake of compound 1 in these cells. The quantification of 1 in the cell culture medium indicated that the cellular uptake was only 8% for this compound (Figure 11E). However, the uptake was slightly higher for compound 2 (15%), which has two chlorine atoms. These experiments provided the initial indications that the halogen atoms play an important role in the cellular uptake. Furthermore, the higher uptake of compound 3 (22%) having two bromine atoms indicated that the introduction of heavier halogen may improve the cellular uptake. Interestingly, a further increase in the uptake was observed upon introduction of an iodine atom, and about 38% uptake was observed for compound 4 (Figure 11D, E). Remarkably, an almost quantitative cellular uptake was observed for the diiodo compound 5 (98%), which suggests that the two larger iodine atoms do not sterically block, but instead they help in the membrane transport. The uptake studies with other mammalian cells such as HEK293 and HeLa showed a similar trend in the transport. The cytotoxicity studies indicated that the presence of heavier halogen atoms does not alter the toxicity of the parent compound.
The 2‐iodo and 2,6‐diiodoanisole moieties in 4 and 5 play an important role in the cellular uptake of these compounds. To understand whether the direct attachment of the methoxyphenyl group to the naphthalimide moiety is crucial for the movement of these molecules across the plasma membrane, a spacer between the fluorophore and 2,6‐diiodoanisole has been introduced and the effect of such modification on the cellular uptake has been studied. For this purpose, the fluorescent properties of compounds 6–11 containing a −CH2−CH2− moiety between the phenyl and naphthalimide groups have been investigated (Figure 12). Although there was a decrease in the fluorescence upon introduction of bromine and

iodine atoms due to heavy‐atom effect, the good fluorescence intensities observed for compounds 6–11 indicated that compounds 6–11 are suitable for the cellular uptake studies (Ungati et al. Chem. Eur. J. 2019, 25, 3391). As expected, a very weak fluorescence was observed for the cells treated with compounds 6 and 7 , and a remarkable enhancement of the fluorescence was observed for cells treated with the diiodo derivative 8. These observations clearly indicate that the presence of two iodine atoms in 8 facilitates the plasma membrane transport as observed earlier for compound 5.
Figure 12. (A) Chemical structures of the naphthalimide‐based fluorescent molecules 6-11. (B) The fluorescence behaviour of 6-11 measured by a plate reader after 30 min of treatment with HepG2 cells. (C) Confocal microscopy images of HepG2 and HeLa cells treated with 6–11 (10 μM) for 30 min (UT: untreated). Scale bar represents 100 μm for HepG2 cells and 50 μm for HeLa cells. (D) Chemical structures of 12–19 . (E) Confocal microscopy images of HepG2 treated with compounds 12 –19 (10 μM ) for 30 min. Scale bar represents 50 μm.
Ref. Ungati et al. Chem. Eur. J. 2019, 25, 3391.
The quantification of compounds entered the cells indicated that the total cellular uptake of compounds 6, 7, and 8 was 12, 32, and 92%, respectively. The remarkably higher uptake of 8 as compared to that of 6 and 7 indicates that the stronger halogen‐bond forming ability of the iodine atoms in 8 plays an important role in the membrane transport. A similar increase in the cellular uptake was observed on moving from 9 (16%) and 10 (38%) to the diiodo derivative 11 (97%), although the replacement of the −NH2 group with an −NMe2 group only marginally improved the cellular uptake. Further studies with other related naphthalimide‐based compounds 12–19 revealed that the uptake of iodine‐containing compounds 15 and 19 is significantly higher than that of their analogues 12–14 and 16–18 , respectively, although the position of the 2,6‐diiodoanisole in 15 and 19 is different from that of compound 5 (Figure 12D,E). These observations indicate that the diiodoanisole and not the napthalimide moiety may act as a recognition unit for the membrane transport.
The Mechanism of Cellular Uptake
It is interesting to note that the biological system uses the larger iodine atoms instead of the relatively smaller chlorine or bromine atoms in the thyroid hormones. As the presence of iodine atoms clearly enhances the cellular uptake of fluorescent molecules, these compounds may enter the cells through one of the transporters of thyroid hormones. When the cellular uptake of compound 5 was studied in the presence of T4 or T3, a significant decrease in the uptake of 5 with an increase in the concentration of T4 or T3 was observed (Figure 13A). Interestingly, a relatively weaker inhibition of cellular uptake was observed in the presence of 3,5‐T2 and there was no inhibition when the uptake studies were carried out in the presence of
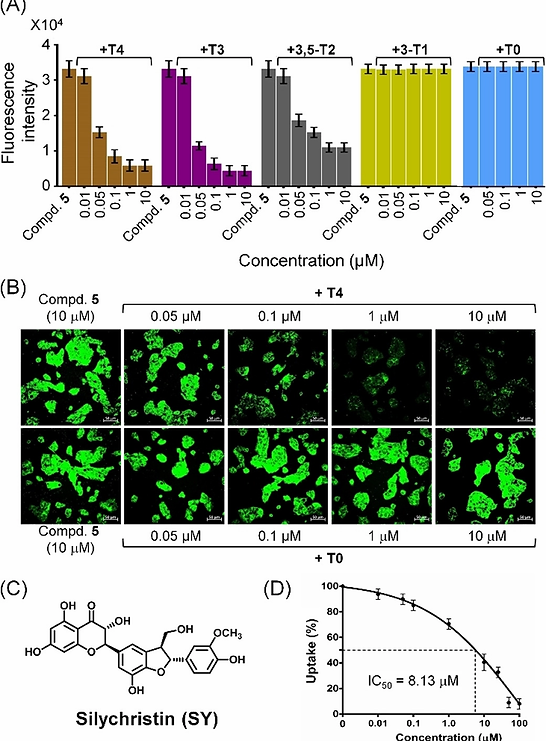
3‐T1 or T0. These observations indicate that compound 5 containing two iodine atoms cannot compete with T4 and T3, that contain four and three iodine atoms, respectively, as the cellular entry of compound 5 is mediated by the same transporter that facilitates the internalization of thyroid hormones. The similar inhibition pattern for the uptake of 5 in the presence of T4 and T3 is consistent with an earlier observation that MCT8 is capable of transporting both T4 and T3 with similar KM values. This observation was further supported by inhibition studies using silychristin, which has been reported as a remarkably specific inhibitor of MCT8. Silychristin inhibited the cellular uptake of compound 5 with an IC50 value of 8.13 μM (Figure 13D). The above study revealed that fluorescent probes and therapeutic compounds can be transported into mammalian cells through the thyroid hormone transporter MCT8 by introducing iodine‐containing chemical moieties.
Figure 13. (A) The fluorescence measured by a plate reader after 30 min of treatment of HepG2 cells with compound 5 (10 μM) in the presence of various concentrations (0.01–10.0 μM) of thyroxine (T4) and its metabolites T3, 3,5‐T2, 3‐T1 and T0. (B) Confocal microscopy images of HepG2 cells co‐treated with 5 (10 μM) and various concentrations of T4 or T0 for 30 min. Scale bar represents 50 μm. (C) Chemical structure of silychristin, a potent inhibitor of the thyroid hormone transporter MCT8. (D) Inhibition of the cellular uptake of 5 by silychristin. The fluorescence was measured by a plate reader after treating the HepG2 cells with various concentrations of silychristin for 20 min, followed by treatment with 5 (10 μM) for 20 min.
Ref. Ungati et al. Angew. Chem. Int. Ed. 2018, 57, 8989.